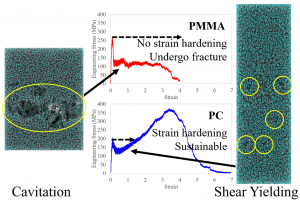
Polymer impact fracture
The study of fractures is one of the most important topics in polymer research. Accordingly, many insightful works about polymer fractures have been reported since the 1960s. In particular, the relationship between molecular motion and fracture strength has been established experimentally, and craze criteria were formulated based on theoretical models. Furthermore, simulations have helped clarify the fracture mechanism at the molecular level. However, despite much research on fractures based on experiments, theoretical models, and calculations, many questions remain unanswered. For instance, the differences in the fracture modes of different materials remain unexplained. Specifically, it is unclear why poly(methyl methacrylate) (PMMA) exhibits brittle fracture while polycarbonate (PC) exhibits ductile fracture. We conducted all-atom MD (AA-MD) simulations of PMMA and PC, which mimic realistic systems, using MODYLAS, which we developed, and the K-computer. Using this approach, we clarified the fracture type differences between PMMA and PC at the molecular level. The figure shows that PMMA exhibits brittleness and PC exhibits ductility.
Solvent cracking
Solvent cracking is a kind of polymer fracture caused by solvents; certain solvents destroy certain polymer materials. Solvent cracking has been studied practically because of its importance for engineering. However, since this is clearly a molecular phenomenon, research at the microscopic level is necessary. We aim to clarify the effect of solvents on polymer failure at the molecular level by studying (1) the dissolution and diffusion behaviors of solvent molecules in polymers and (2) the response of solvent molecules during polymer failure.
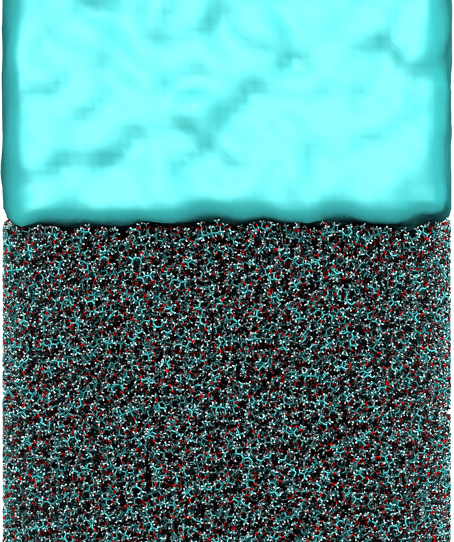
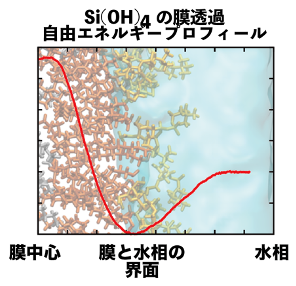
Membrane transport of silicic acid hydrate
The use of inorganic elemental materials in appropriate concentrations to induce tissue regeneration is widely reported. Silicic acid hydrate in a wide range of shapes and sizes promotes osteoblast osteodifferentiation, and is a beneficial inorganic elemental material among many candidates. The uptake of nanoparticles (about 60 nm in diameter) composed mainly of silicate hydrate has been reported to proceed via pinocytosis. On the other hand, the uptake mechanism of silicate hydrates smaller than 10 nm, which are more effective in inducing tissue regeneration than nanoparticles, is unknown. This study aims to elucidate the absorption mechanism of silicate hydrates at the molecular level by taking advantage of MD calculations. The uptake of silicate hydrate occurs via pinocytosis, which involves the uptake of extracellular fluid (the aqueous solution around the cell), or passive transport, which involves the passage of silicate hydrate through the membrane due to thermal motion. In the case of pinocytosis, substances that have a low cell adsorption free energy and, therefore, tend to collect around the cell are easily taken up. In the case of passive transport, substances with a high membrane permeability, i.e., the number of molecules that permeate per unit area per unit time, are more likely to be taken up. Therefore, the mechanism will be clarified by estimating the cell adsorption free energy and membrane permeability of silicate hydrates.
Solubilization
When amphiphilic surfactants are dissolved in aqueous solutions above the critical micelle concentration, they form spherical micelles with hydrophobic groups on the inside and hydrophilic groups on the outside. When such spherical micelles exist in aqueous solutions, insoluble substances such as octane may be incorporated into the micelles and dispersed in water. This phenomenon is called solubilization. Solubilization has a wide range of applications, from industrial products to pharmaceuticals, including inks, cosmetics, and medicines. Although this phenomenon has a variety of applications, it is difficult to answer questions such as “Why are solubilized molecules incorporated into micelles?”, “How many molecules are incorporated?”, and “Where in the micelle are the solubilized molecules incorporated?”. These questions have not been explored through molecular-level studies. Consequently, we conducted AA-MD simulations to calculate (1) the free energy difference between aqueous solubilized molecules and micelles for simple linear alkanes and molecules with typical hydrophilic groups (methylamine, octylamine, methanol, and octanol), and (2) the free energy profile using the distance between the micelles and solubilized molecules as the reaction coordinate. Our simulations showed that hydrophobic interactions were a driving force for micelle solubilization.
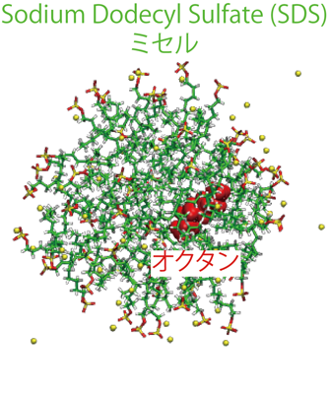